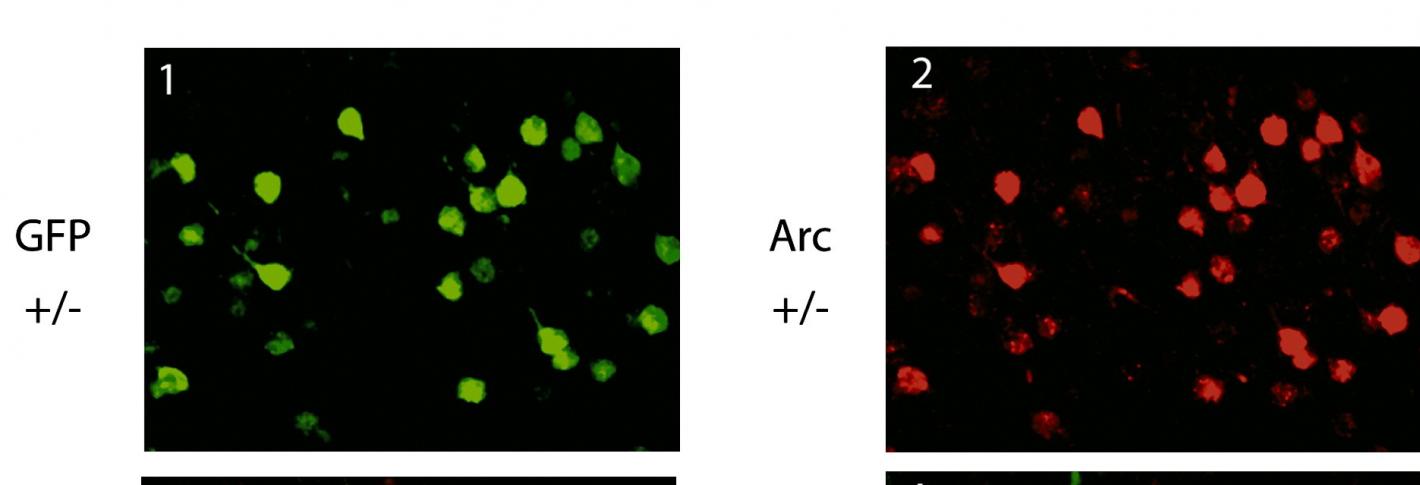
Memories form when cells connect into ensembles called engrams. This ability of neurons to change their connections, called synapses, in response to experience is called “synaptic plasticity.” In several studies, including ones dating back a decade before the Picower Institute began, the lab of Susumu Tonegawa has identified crucial genetic and molecular underpinnings of the synaptic plasticity of memory. Below are just a few examples of key discoveries in this area of research in the lab.
In 1992 Tonegawa’s lab demonstrated for the first time that genes for synaptic plasticity were crucial for memory. His lab knocked out the αCaMKII gene and showed that doing so impaired spatial memory. Four years later his team highlighted another genetic mechanism of synaptic plasticity by knocking it out in specific neurons in a key region for memory in the brain, the hippocampus.
With that background in 2002, the first year of The Picower Institute, Tonegawa and colleagues further showed that a gene for the NMDA receptor, a crucial component of synaptic plasticity, was necessary in hippocampus area CA3 for mice to be able recall a full memory when only given incomplete cues (a mental function called pattern completion). Five years later they knocked out NMDA receptors in granule cells of the dentate gyrus area of the hippocampus, discovering that they were crucial for pattern separation, which is the ability to distinguish between similar contexts.
Above: Neurons stained both with green fluorescent protein and also (right) for expression of Arc.
To illuminate the phenomenon of neuroplasticity, the ability of neurons to change their physiology in response to experience-driven activity, his lab in 2006 developed a technology to visualize it in action by fluorescently tagging the protein Arc so that its expression could be monitored via two-photon microscopy as mice gained experience with visual stimuli. This pioneering study demonstrated a new ability to directly visualize the molecular activity of individual neurons in the brains of live animals at a single-cell resolution, and to observe the changes in the activity in the same neurons in response to the changes of the environment on a daily basis for days at a time.
And in 2008 the lab produced a powerful tool for inducibly and reversibly disrupting synaptic transmission in hippocampal circuits to determine their function. The innovative study showed that a long circuit connecting the entorhinal cortex, through several regions of the hippocampus and back was necessary for multiple forms of learning but that a simpler form of spatial learning could be achieved without the circuit if a simpler one was left undisrupted.